ANN ARBOR — In findings that could change the way industries like nuclear energy and aerospace look for materials that can stand up to radiation exposure, University of Michigan researchers have discovered that metal alloys with three or more elements in equal concentrations can be remarkably resistant to radiation-induced swelling.
The big problem faced by metals bombarded with radiation at high temperatures—such as the metals that make up nuclear fuel cladding—is that they have a tendency to swell up significantly. They can even double in size.
“First, it may interfere with other parts in the structure, but also when it swells, the strength of the material changes. The material density drops,” said Lumin Wang, U-M professor of nuclear engineering and radiological sciences. “It may become soft at high temperatures or harden at low temperatures.”
This happens because when a particle flies into the metal and knocks an atom out of the crystal structure, that displaced atom can travel quickly through the metallic crystal. Meanwhile, the empty space left behind doesn’t move very fast. If many atoms are ousted from the same area, those empty spaces can coalesce into sizable cavities.
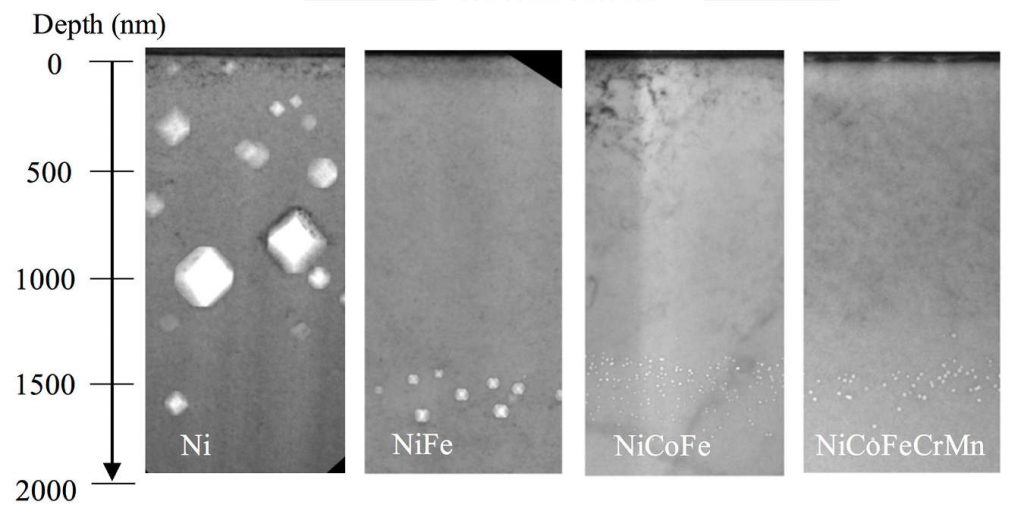
An electron microscope reveals the radiation-induced cavities inside samples of pure nickel and alloys. The cavities in nickel-cobalt-iron and nickel-cobalt-iron-chromium-manganese alloys are 100 times smaller than those in pure nickel. Image credit: Wang Group, University of Michigan (Click image to enlarge)
To control the formation of these cavities, and the attendant swelling, most recent research has focused on creating micro- and nano-structures inside the metal as specially designed “sinks” to absorb small defects in a way that preserves the integrity of the material. But Wang and his colleagues are kicking it old school, looking at alloys that don’t have breaks in the crystal structure of the atoms.
Colleagues at Oak Ridge National Laboratory in Tennessee created samples of a variety of nickel-based alloys. These were then exposed to radiation in a facility at the University of Tennessee. The most successful alloys were concentrated solid solutions—crystals made of equal parts nickel, cobalt and iron; or nickel, cobalt, iron, chromium and manganese.
“These materials have many good properties such as strength and ductility, and now we can add radiation tolerance,” said Chenyang Lu, a U-M postdoctoral research fellow in nuclear engineering and radiological sciences and the leading author of the report in Nature Communications.
In an experiment proposed by Wang, UT researchers exposed the samples to beams of radiation that created two levels of damage, similar to what may accumulate in a reactor core over several years and over several decades. These experiments were done at a temperature of 500 Celsius or 932 Fahrenheit—a temperature at which nickel-based alloys are usually prone to swelling.
These samples were analyzed at U-M’s Center for Material Characterization with a transmission electron microscope. The team found that compared to pure nickel, the best alloys had more than 100 times less radiation damage.
To explain what was special about these alloys, the team worked closely with the group of Fei Gao, a theoretician and U-M professor of nuclear engineering and radiological sciences. Gao’s group performed computer simulations at the level of individual atoms and showed that the radiation tolerance in this group of alloys can be attributed to the way that the displaced atoms travel within the material. The explanation was further confirmed by another set of experiments conducted by the team at the University of Wisconsin.
“In simplified terms, if there are a lot of atoms of different sizes, you can consider them bumps or potholes,” Wang said. “So this defect won’t travel so smoothly. It will bounce around and slow down.”
Because the displaced atoms and the holes in the crystal structure stayed near one another, they were much more likely to find one another. In effect, this repaired many of the vacancies in the complicated alloys before they could join together into larger cavities.
“Based on this study, we now understand how to develop a radiation-tolerant matrix of an alloy,” Wang said.
The study, titled “Enhancing radiation tolerance by controlling defect mobility and migration pathways in multicomponent single phase alloys,” appears in Nature Communications.
The work was supported as part of the Energy Dissipation to Defect Evolution Center, an Energy Frontier Research Center funded by the U.S. Department of Energy, Office of Science, Basic Energy Sciences.
*Source: University of Michigan