— Scientists use special kind of atomic force microscopy at low temperatures and in ultrahigh vacuum to image the charge distribution within a single molecule
— The new technique will further the understanding of nanoscale physics and could help develop future applications such as solar photoconversion, energy storage, or molecular scale computing devices
ZURICH – 27 Feb 2012: IBM scientists were able to measure for the first time how charge is distributed within a single molecule. This breakthrough will enable fundamental scientific insights into single-molecule switching and bond formation between atoms and molecules. The ability to image the charge distribution within functional molecular structures holds great promise for future applications such as solar photoconversion, energy storage, or molecular scale computing devices.
As reported recently in the journal Nature Nanotechnology, scientists Fabian Mohn, Leo Gross, Nikolaj Moll and Gerhard Meyer of IBM Research succeeded in imaging the charge distribution within a single molecule by using a special kind of atomic force microscopy called Kelvin probe force microscopy at low temperatures and in ultrahigh vacuum.
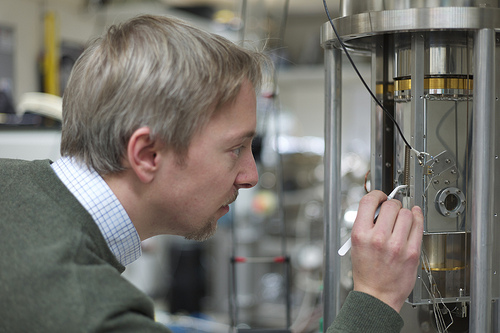
Fabian Mohn of the Physics of Nanoscale Systems group at IBM Research – Zurich. As reported in in the journal Nature Nanotechnology, scientists Fabian Mohn, Leo Gross, Nikolaj Moll and Gerhard Meyer of IBM Research – Zurich directly imaged the charge distribution within a single naphthalocyanine molecule using a special kind of atomic force microscopy called Kelvin probe force microscopy at low temperatures and in ultrahigh vacuum. Image credit: IBM
“This work demonstrates an important new capability of being able to directly measure how charge arranges itself within an individual molecule,” states Michael Crommie, Professor in the Department of Physics at the University of California, Berkeley. “Understanding this kind of charge distribution is critical for understanding how molecules work in different environments. I expect this technique to have an especially important future impact on the many areas where physics, chemistry, and biology intersect.”
The new technique provides complementary information about the molecule, showing different properties of interest. This is reminiscent of medical imaging techniques such as X-ray, MRI, or ultrasonography, which yield complementary information about a person’s anatomy and health condition.
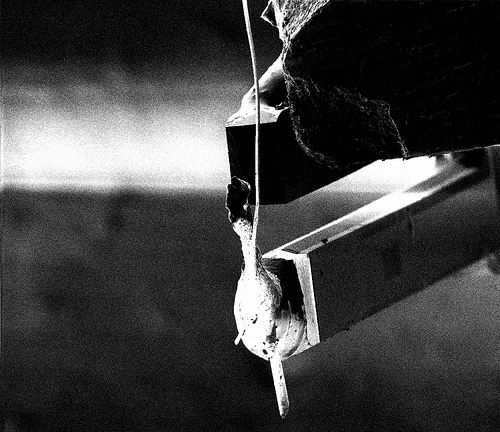
For their experiments the IBM scientists used their home-built combined scanning tunneling microscope (STM) and atomic force microscope (AFM). In this focused ion beam micrograph, the tip attached to a tuning fork can be seen. The tuning fork measures a few millimeters in length. The tiny tip measures only a single atom or molecule at its apex. Image credit: IBM
The discovery could be used to study charge separation and charge transport in so-called charge-transfer complexes. These consist of two or more molecules and hold tremendous promise for applications such as computing, energy storage or photovoltaics. In particular, the technique could contribute to the design of molecular-sized transistors that enable more energy efficient computing devices ranging from sensors to mobile phones to supercomputers.
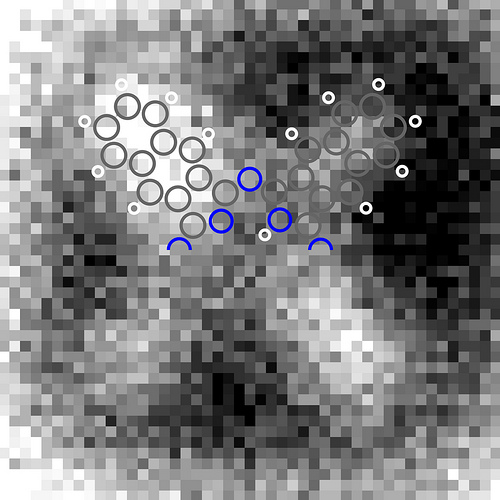
Kelvin probe force microscopy images of the tautomerization switching of naphthalocyanine. It is the fist time that the charge distribution within a single molecule can be resolved. When a scanning probe tip is placed above a conductive sample, an electric field is generated due to the different electrical potentials of the tip and the sample. With KPFM this potential difference can be measured by applying a voltage such that the electric field is compensated. Therefore, KPFM does not measure the electric charge in the molecule directly, but rather the electric field generated by this charge. The field is stronger above areas of the molecule that are charged, leading to a greater KPFM signal. Furthermore, oppositely charged areas yield a different contrast because the direction of the electric field is reversed. This leads to the light and dark areas in the micrograph. Image courtesy of IBM Research – Zurich
“This technique provides another channel of information that will further our understanding of nanoscale physics. It will now be possible to investigate at the single-molecule level how charge is redistributed when individual chemical bonds are formed between atoms and molecules on surfaces,” explains Fabian Mohn of the Physics of Nanoscale Systems group at IBM Research – Zurich. “This is essential as we seek to build atomic and molecular scale devices.”
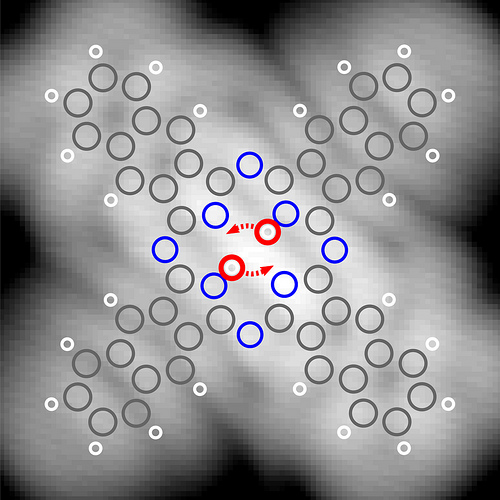
Scanning tunneling microscopy (STM) imaging of naphthalocyanine on an ultrathin layer of natrium chloride on copper. STM constant-current image of a naphthalocyanine molecule, recorded with a CO-terminated tip. The DFT-calculated atomic positions in the molecule are overlaid, with carbon, hydrogen and nitrogen atoms in grey, white and blue, respectively. The positions of the central hydrogens and the tautomerization path are highlighted in red. Image courtesy of IBM Research – Zurich
Gerhard Meyer, a senior IBM scientist who leads the scanning tunneling microscopy (STM) and atomic force microscopy (AFM) research activities at IBM Research – Zurich adds, “The present work marks an important step in our long term effort on controlling and exploring molecular systems at the atomic scale with scanning probe microscopy.”
For his outstanding work in the field, Meyer recently received a European Research Council Advanced Grant. These prestigious grants support “the very best researchers working at the frontiers of knowledge” in Europe.*
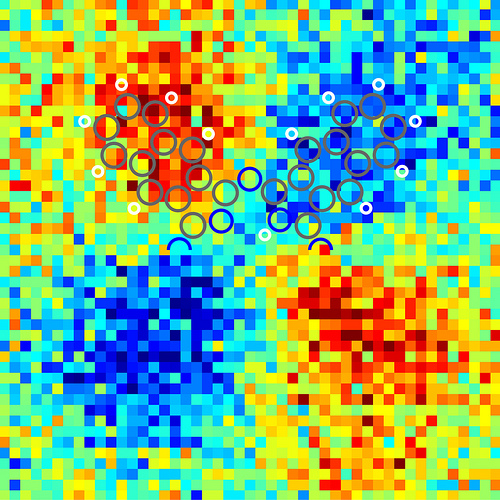
Asymmetry in the Kelvin probe force microscopy images of the tautomerization switching of naphthalocyanine. It is the first time that the charge distribution within a single molecule can be resolved. When a scanning probe tip is placed above a conductive sample, an electric field is generated due to the different electrical potentials of the tip and the sample. With KPFM this potential difference can be measured by applying a voltage such that the electric field is compensated. Therefore, KPFM does not measure the electric charge in the molecule directly, but rather the electric field generated by this charge. The field is stronger above areas of the molecule that are charged, leading to a greater KPFM signal. Furthermore, oppositely charged areas yield a different contrast because the direction of the electric field is reversed. This leads to the red and blue areas in the micrograph. Image courtesy of IBM Research – Zurich
Taking a closer look
To measure the charge distribution, IBM scientists used an offspring of AFM called Kelvin probe force microscopy (KPFM).
When a scanning probe tip is placed above a conductive sample, an electric field is generated due to the different electrical potentials of the tip and the sample. With KPFM this potential difference can be measured by applying a voltage such that the electric field is compensated. Therefore, KPFM does not measure the electric charge in the molecule directly, but rather the electric field generated by this charge. The field is stronger above areas of the molecule that are charged, leading to a greater KPFM signal. Furthermore, oppositely charged areas yield a different contrast because the direction of the electric field is reversed. This leads to the light and dark areas in the micrograph (or red and blue areas in colored ones).
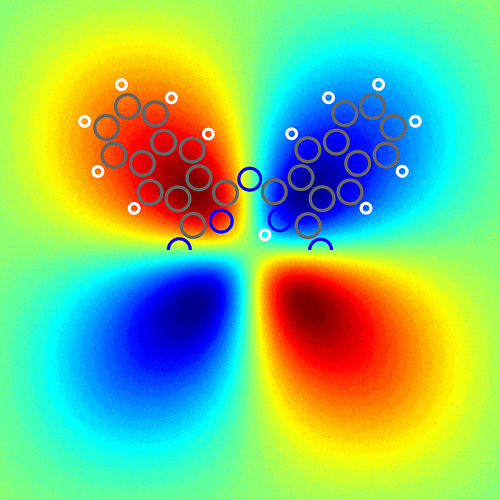
Density-functional theory calculation of the asymmetry of the electric field above a free naphthalocyanine molecule at a distance d = 0.5 nanometers from the molecular plane. Image courtesy of IBM Research – Zurich
Naphthalocyanine, a cross-shaped symmetric organic molecule which was also used in IBM’s single-molecule logic switch**, was found to be an ideal candidate for this study. It features two hydrogen atoms opposing each other in the center of a molecule measuring only two nanometers in size. The hydrogen atoms can be switched controllably between two different configurations by applying a voltage pulse. This so-called tautomerization affects the charge distribution in the molecule, which redistributes itself between opposing legs of the molecules as the hydrogen atoms switch their locations.
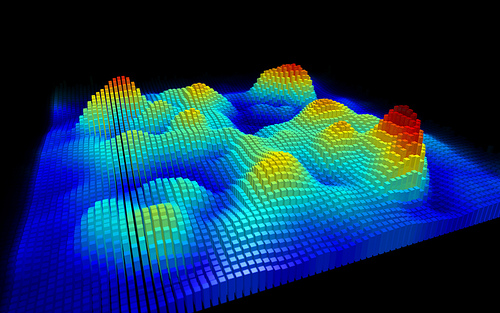
3D representation of the experimental Kelvin probe force microscopy image of a single naphthalocyanine molecule, recorded with a CO-functionalized tip. Image courtesy of IBM Research – Zurich
Using KPFM, the scientists managed to image the different charge distributions for the two states. To achieve submolecular resolution, a high degree of thermal and mechanical stability and atomic precision of the instrument was required over the course of the experiment, which lasted several days.
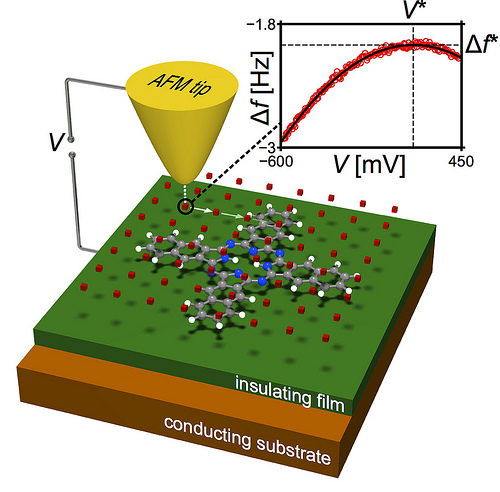
Schematic of the measurement principle. At each tip position, the frequency shift is recorded as a function of the sample bias voltage (inset, red circles). The maximum of the fitted parabola (inset, solid black line) yields the KPFM signal V* for that position. Image courtesy of IBM Research – Zurich
Moreover, adding just a single carbon monoxide molecule to the apex of the tip enhanced the resolution greatly. In 2009, the team has already shown that this modification of the tip allowed them to resolve the chemical structures of molecules with AFM. The present experimental findings were corroborated by first-principle density functional theory calculations done by Fabian Mohn together with Nikolaj Moll of the Computational Sciences group at IBM Research – Zurich.
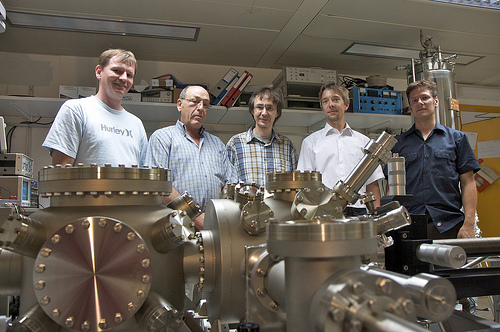
IBM Research – Zurich scientists Nikolaj Moll, Reto Schlittler, Gerhard Meyer, Fabian Mohn and Leo Gross (left to right) standing behind an scanning tunneling/atomic force microscope similar to the one they used to image the “anatomy” of the Pentacene molecule at an atomic resolution. Photo taken by Michael Lowry. Image courtesy of IBM Research – Zurich
The scientific paper entitled “Imaging the charge distribution within a single molecule” by F. Mohn, L. Gross, N. Moll, and G. Meyer was published online in Nature Nanotechnology, DOI: 10.1038/NNANO.2012.20 (26 February 2012).
* cited from the ERC press release, January 24, 2012: https://erc.europa.eu/sites/default/files/press_release/files/press_release_adg2011_results.pdf
** P. Liljeroth, J. Repp, and G. Meyer, “Current-Induced Hydrogen Tautomerization and Conductance Switching of Naphthalocyanine Molecules”, Science 317, p.1203–1206 (2007), DOI: 10.1126/science.1144366
*Source: IBM